Research
The Henninger lab explores mechanisms by which RNA regulates cell fate in health and disease. Active gene regions are crowded with RNA molecules that can participate in gene regulation. These RNA molecules can mediate molecular interactions and act as scaffolds or regulators of large assemblies of proteins involved in transcription and RNA processing. Our lab uses a combination of molecular biology, soft-matter physics, and super-resolution imaging to investigate how RNA regulates genes and contributes to cell fate. We aspire to uncover fundamental mechanisms solving mysteries in gene control, inspiring the development of new RNA-based therapies, and helping us understand how RNA defects contribute to human disease.
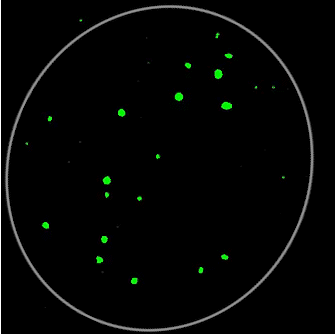
Super-resolution imaging of transcriptional condensates in a mouse embryonic stem cell.
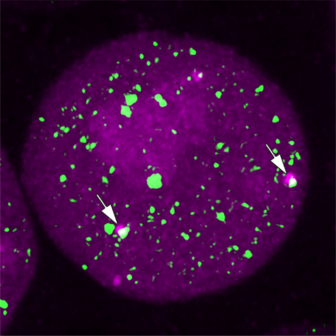
Super-resolution imaging of transcriptional condensates (green) and the production of nascent RNA (magenta) at two alleles (arrows).
RNA in transcriptional condensates
Condensates are large assemblies formed by interactions between biopolymers and are subject to thermodynamic parameters such as concentration of constituents, the valency of interactions, temperature, and solvent properties. RNA molecules are powerful regulators of condensates, acting as scaffolds of condensate assembly and altering their physicochemical properties.
Transcriptional proteins, DNA, and RNA form transcriptional condensates at active genes. We previously discovered that during early stages of transcription, RNA can stimulate condensate formation, while bursts of RNA synthesis that occur during transcription elongation can induce condensate dissolution. This simple but powerful mechanism of feedback control allows RNA to mediate its own transcription.
Our lab seeks to further understand how RNA impacts transcriptional and co-transcriptional RNA processing condensates during gene activation.
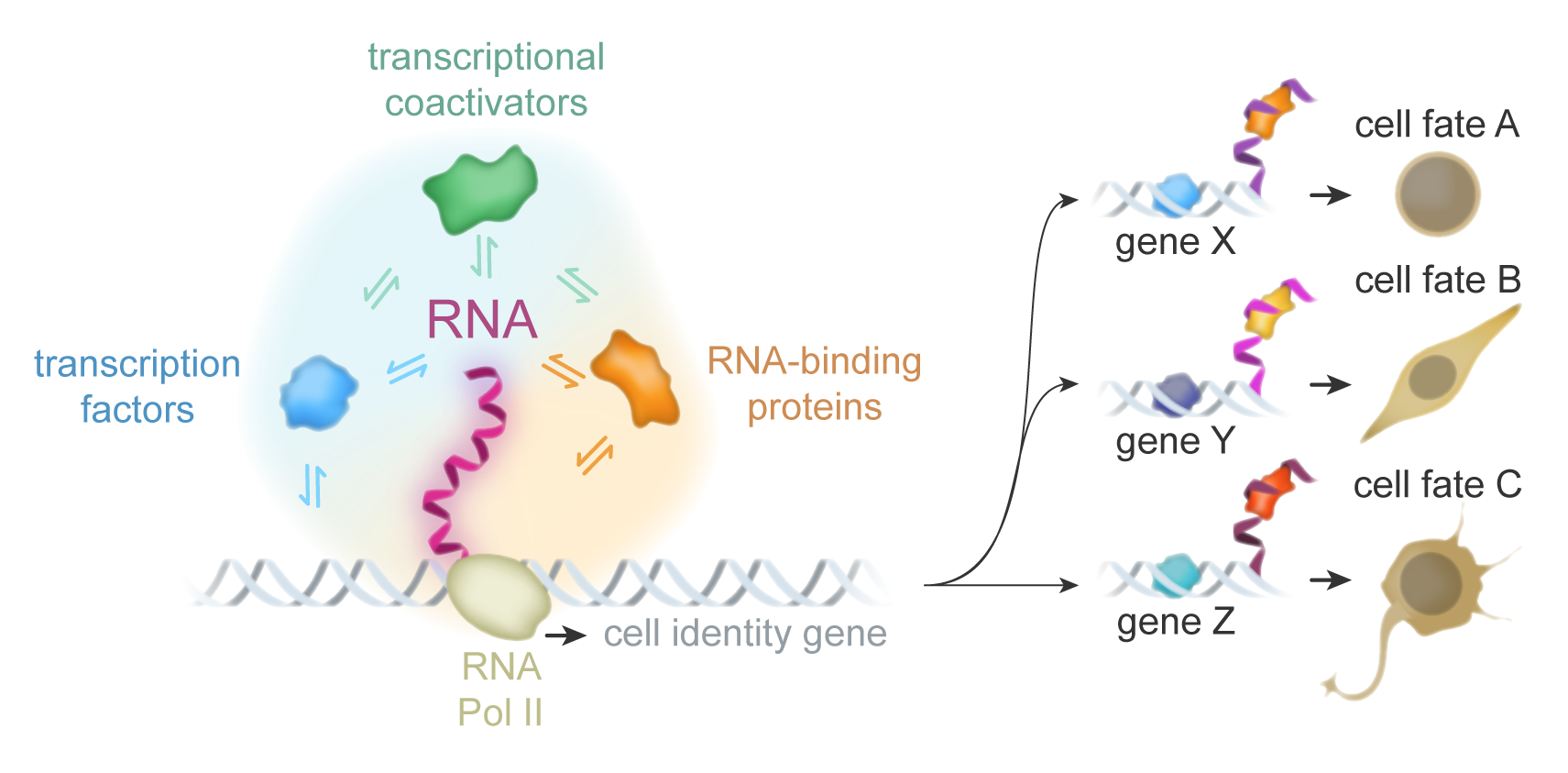
RNA-encoded gene regulation and cell fate
Foundational models of transcriptional regulation involve the assembly of protein complexes at DNA elements associated with specific genes. These assemblies include transcription factors, cofactors, RNA polymerase, and chromatin regulators. This protein-centric view has been altered with recent evidence that many of these proteins also bind RNA, and RNA molecules have important roles to play in gene regulation.
Our lab seeks to understand how RNA molecules contribute to the activation and repression of genes involved in differentiation and cell fate, with the goal of decoding an RNA-based cis regulatory code.
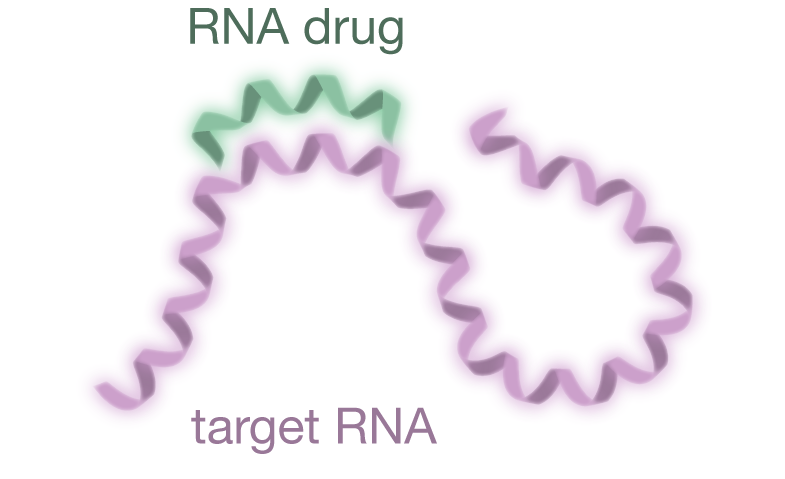
RNA-based therapeutics and RNA defects in disease
RNA-based drugs have emerged as a new method to address a variety of diseases, although significant challenges remain. RNAs are difficult to deliver to many tissues and subcellular locations. But these molecules hold promise to tackle therapeutic challenges that have remained resilient to conventional small molecule drugs, including the ability to target the expression of genes. Our lab explores methods to enhance subcellular targeting of RNA-based drugs, and investigates the ability of RNA to modestly stimulate or repress expression of disease-associated genes for therapeutic benefit.
Many diseases are caused by mutation of RNA-binding proteins. For instance, mutations in multiple RNA-processing factors (e.g. SRSF2, SF3B1, U2AF1, ZRSR2) can contribute to the pathogenesis of acute myeloid leukemia. Our lab seeks to explore the mechanisms by which these mutated factors contribute to cancer development.
The genome revolution has provided a wealth of data on human genetic variants associated with traits or linked to disease. The vast majority of these variants are not in protein-coding sequence but instead exist in gene regulatory regions. Our lab explores the possibility that a subset of these variants disrupt RNA, rather than DNA, function.